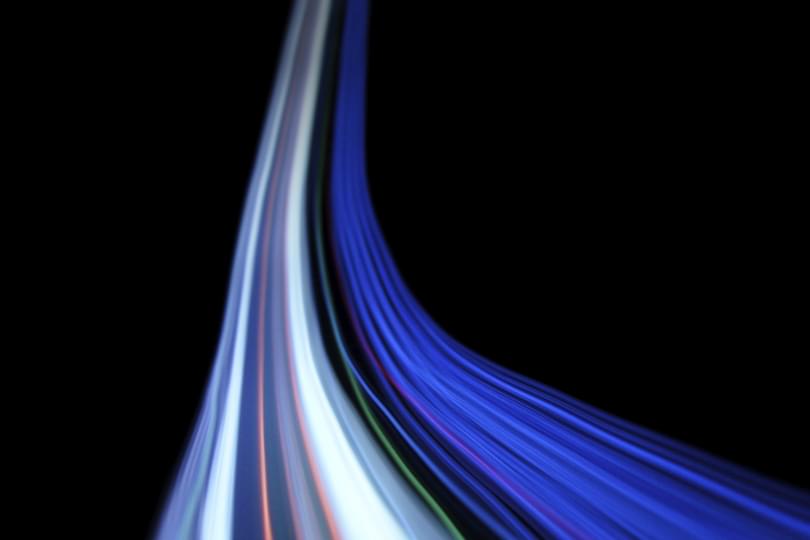
Quantum computers exist as blueprints on paper, waiting to be turned into real, physical, machines. To give you an idea of the power of such information processors, think of the difference between today's computers and the first pocket calculators. They aren’t just going to be much faster, that’s a given, but they’ll open up whole new kinds of problems that today’s computers can’t begin to tackle. That’s much more significant than speed.
And the reason for this might seem a little subtle and arcane, but is actually quite straightforward if you image the following. Imagine asking a classical computer to model, say, an ecosystem in a forest. This includes trees, birds and bugs. Now if you double the number of trees, birds and bugs in your ecosystem, the time the computer will take to run through all the different interactions in the ecosystem twice the size, won’t just double, but will increase to the power two. And if you triple this, it’ll be to the power three, and so on.
Very quickly indeed you run out of computing power, so in the end, our real-world computer models of, say, the climate, look like toy models giving only rough impressions of what is happening. And that's even using today’s most advanced supercomputers.
The punch line is this: if you used a quantum computer instead, the time it would take you to do the same calculation would only increase twice, or three times rather than to the power two or three, as you double or triple the number of trees, birds and bugs in your model. If you’ve understood this, you’ve understood what several Nobel prizes were awarded for.
It’s a powerful idea when you think of all the exciting breakthroughs that could be achieved with quantum computers, such as new drugs against HIV or cancer designed using quantum computers to simulate chemical interactions at the molecular level, which would end the lengthy trial and error processes that push up costs for today’s drug manufacturers.
So what are scientists waiting for to make quantum computers real?
The physics is pretty well understood, and everything suggests that nothing in the fundamental physical laws governing quantum theory prevents us from making a quantum computer today.
The challenge is an engineering one. And it’s a formidable one at that because we’re talking about designing and manipulating objects at the nanoscale – that’s one billionth of a metre. At this scale, objects behave in the strange ways stipulated by the laws of the very small; quantum laws, where particles like electrons are allowed to leak through walls, enter two doors at the same time, and so on. Marshalling such wildly behaved particles to get them to carry the information you encode into them poses some serious challenges.
The biggest challenge is to insulate the particles from heat in surroundings while you manipulate them. That’s an almost self-contradictory mission, yet it’s what thousands of physicists around the world are racing to crack. Heat in our surroundings transfers to the particles as energy, which erases the information we want the particles to store. So next time you come across a scientific paper by a quantum physicist, you can be confident that it will be describing an advance in one form or another on the durability of information stored in a particle.
In the scientific jargon, information storage in a particle is called a quantum state – think of it as a shelf with one piece of information on it, like a book, if you will. While the word “quantum” just means that the shelf can only hold an integer number of pieces of information, rather than fractional amounts, but that’s unimportant for what follows.
Ten years ago, a string of fascinating reports began to trickle out of laboratories studying photosynthetic molecules – these specialise in converting light into chemical energy. Their claim was quite astounding, because they said that quantum computers could already be all around us, in the form of leaves on trees and photosynthetic bacteria living deep in oceans. These survive by harvesting particles of light that they converted into particles of energy on capture, and which then have to find their way through a tangled web of paths through the molecule leading to a so-called chemical reaction centre. Only on reaching the centre can the organism feed.
All these reports said that the particle of energy found its way to the reaction centre so fast and without ever getting lost, that this necessarily meant that that a quantum computation had been performed to allow the particle to find the shortest path to its destination.
How does that work? Here the reader is asked to briefly suspend disbelief. The particle superposes itself over possible paths to the reaction centre, “sniffs out” the shortest, then goes down that route. The notion of superposition sounds a bit fantastical, but describes the real ability of particles to “smudge” themselves over several places and to go through two “doors” at the same time. The phenomenon is routinely observed in experiments but its interpretation remains the subject of philosophical debate and physicists’ dinner parties…
But let’s get back to quantum-assisted photosynthesis. If true, the inspiration from natural systems could yield the breakthrough needed for an artificial quantum processor. We could then copy natural systems in artificial designs that would similarly keep quantum states alive just long enough for calculations to take place.
The idea that some natural systems could use quantum computation as a tool to help them function and even survive isn’t completely implausible. A bolder claim was made for biological organisms in general by no lesser luminaries than the founders of quantum physics themselves, Schroedinger and Heisenberg, who famously likened the biologists of their day to kids playing with “balls and sticks” models, which won’t do, they said.
It’s not implausible to argue that under the action of evolution over billions of years, nature could have evolved systems capable of maintaining quantum states just long enough to allow certain quantum computations to happen. For a bacterium sitting at the bottom of a deep dark ocean where it ekes out a living on a diet of one or two photons a day, quantum computation-assisted photosynthesis could mean the difference between life and death.
This opinion piece reflects the views of the author, and does not necessarily reflect the position of the Oxford Martin School or the University of Oxford. Any errors or omissions are those of the author.